USING PREDICTIVE MONITORING TO MITIGATE CONSTRUCTION IMPACTS IN SENSITIVE MARINE ENVIRONMENTS
Riku W. Koskela1, Petra Ringeltaube1, Andrew R. Small1, Thomas V. Koskela1, Angus R. Fraser1, Joanna D. Lee1 and Paul Marshall2
1Aquatic Sciences Group, GHD Pty Ltd
Brisbane, Queensland, AUSTRALIA
rkoskela@ghd.com.au
2Monitoring and Assessment Unit, Great Barrier Reef Marine Park Authority
Townsville, Queensland, AUSTRALIA
ABSTRACT
A Predictive Monitoring Program (PMP) has been developed to mitigate construction
related impacts in sensitive marine environments. The program aimed to intervene in the pollutant-generating
process before the occurrence of environmental impacts. This has been achieved through compliance monitoring
of the key pollutant processes using process specific trigger values. These trigger values were derived
from a hydrodynamic model that incorporated habitat or biota tolerances to the pollutant. The Predictive
Monitoring Program has been effectively implemented to mitigate the impacts of two common construction
processes, these being de-watering discharge (Nelly Bay Safe Harbour Development, Magnetic Island, Townsville,
Australia) and cutter-suction dredging (East Arm Port Stage 2A, Darwin, Australia) on nearby coral and
mangrove communities ( Great Barrier Reef Marine Park and Charles Darwin National
Park respectively). This program differs substantially from the conventional Environmental Impact
Monitoring Program, which requires the occurrence of measurable environmental harm prior to implementation
of a management response.
INTRODUCTION
Substantial engineering works including port construction, dredging, coastal
stabilisation, reclamation and wastewater discharge are occasionally undertaken within or in close proximity
to marine protected areas. Authorities seek to moderate the impact of such works through various regulatory
tools, management frameworks and environmental obligations ( Craik, 1996; Puckette,
1998). As part of this mitigation process, many authorities require the design and implementation
of an Impact Monitoring Program (IMP). The conventional IMP seeks to identify environmental impacts that
result from a construction or pollutant-generating process. This includes routine monitoring of receiving
water quality and benthic substrates ( Jorgensen and Edelvang, 2000; Elander
and Hammar, 1998; Ruffin, 1998; Wolanski and Gibbs, 1992), and in some instances, condition assessments
of key habitats or biota ( Healy et al., 1999; Quigley and Hall, 1999; Marques
et al., 1993; Nichols et al., 1990; Lawing and Hanumara, 1989). However, using impact assessment of
key habitats as the primary trigger for management response is a somewhat self-defeating approach to environmental
protection. This is because measurable environmental harm must be demonstrated before it can be recognized
that mitigation measures are required.
Clearly, it is desirable to intervene in the construction or pollutant-generating
process prior to the occurrence of a measurable impact in the receiving environment. Gray
and Jensen (1993) identified the need for a marine monitoring system that integrated construction
effects and ecosystem condition to allow for rapid management response. Bach et
al. (1997) applied this concept in their Feedback Monitoring System. The system was developed to protect
an eelgrass community from increased turbidity during dredging and reclamation works. The monitoring program
was developed by integrating a mathematical model of turbidity with known turbidity tolerances of eelgrass
to minimise physiological stress during construction. In this instance, eelgrass condition was the key
performance indicator, however a lag time between impact and management response was in the order of 2
weeks (Bach et al., 1997).
More recently, Clarke and Wilbur (2000) have
highlighted the need to integrate biological and engineering information to objectively evaluate potential
environmental impacts. The ability to do this has been enhanced by ongoing advances in hydrodynamic modelling
( Botev and Fryar, 2002) combined with improved understanding of biota and
habitat tolerances to various pollutants. This approach has been integrated in a Predictive Monitoring
Program (PMP) that has been designed for the protection of sensitive environments and implemented for
the protection of a coral and a mangrove community during two major construction projects. These projects
were undertaken in the Great Barrier Reef Marine Park (Nelly Bay, Magnetic Island, Queensland, Australia)
and adjacent to the Charles Darwin National Park (East Arm Port, Darwin Harbour, Northern Territory, Australia).
This paper presents the overall philosophy of a PMP by discussing the outcomes of the two cases studies. In particular, it will describe how the program can substantially reduce the likelihood of construction impacts in sensitive marine environments. This has been achieved by focusing monitoring primarily on construction related pollutant-generating processes to instigate rapid management response. Condition assessment of the receiving habitats (sensitive environments) was used to validate the efficacy of the program.
MATERIALS & METHODS
Predictive Monitoring Program
Design and implementation of the PMPs required a staged process including pre-construction consultation, baseline data collection and hydrodynamic modelling. A schematic overview of this process is presented in Figure 1. The stages in this process include:
1. Identification of key habitats, biota and buffer zones through field assessment and consultation with stakeholders and regulatory authorities.
2. Identification of pollutant-generating construction processes and those pollutants that present a risk to the key habitats or biota. This enables the PMP to focus on the principal pollutants and the construction processes that generate them.
3. Conduct baseline studies on both key habitat or biota condition and natural variability of the principal pollutants with the system.
4. Determination of the tolerances of key habitats or biota to the principal pollutants through specialist review and baseline studies.
5. Development of a hydrodynamic model of the pollutant-generating process that integrates habitat tolerances, mixing and dispersion, tidal currents, sea state and buffer zones. The output of this model is a trigger value, which is the concentration of the pollutant that can be generated by the construction process without exceeding the key habitat tolerance.
6. Undertake validation studies of model outputs to allow for refinement of the trigger value.
7. Undertake compliance-monitoring of the construction process.
8. Instigate immediate pre-defined management actions in response to non-compliance.
9. Undertake routine biophysical monitoring of key habitats to validate the effectiveness of the PMP.
(Enlarge: 23KB) |
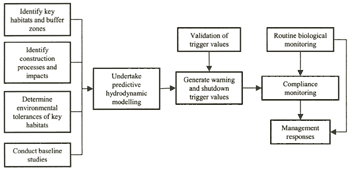 |
Figure 1. Schematic diagram showing the steps involved in the design of
a Predictive Monitoring Program
Case studies
Predictive Monitoring Programs have been applied to two common pollutant-generating construction processes, these being de-watering discharge during construction of Nelly Bay Safe Harbour (Fig.2a) and cutter-suction dredging during East Arm Port extensions (Fig.2b). Detailed description of technical methods will be published elsewhere. The following represents an overview of these two monitoring programs.
Figure 2 (a). Aerial view of Nelly Bay Safe Harbour construction site, and
(b) East Arm Port construction site.
Pre-discharge Monitoring - Nelly Bay Safe Harbour
Nelly Bay Safe Harbour is being constructed on Magnetic Island (19°08 S, 146°50 E), within the Great Barrier Reef Marine Park.
Key habitats: Protected habitats within the development area include fringing platform coral reefs located adjacent to the harbour breakwater and harbour entrance channel. These reef communities were selected as key habitats.
Pollutant-generating construction process: The harbour was sealed by
a temporary semi-permeable breakwater and de-watering occurred at a discharge rate of 〜5000 m 3.hr -1.
This water was pumped to a discharge point 100 m offshore. De-watering of sediment-laden waters from the
harbour basin was a principal pollutant-generating construction process. As light attenuation and sediment
deposition is known to substantially impact coral reef systems, suspended sediment was identified as the
key pollutant ( Loya, 1976; Hodgson, 1989; Rogers, 1990; Perry, 1996). For the
purpose of this monitoring program, turbidity (ntu) was used as a surrogate measure of suspended sediment.
The ability to monitor water quality prior to discharge enabled predictive monitoring for Nelly Bay to
take the form of a pre-discharge monitoring program.
Baseline monitoring: To determine the natural variability of turbidity (ntu), baseline surveys were undertaken through the deployment of in situ turbidity loggers at various reef slope stations. Baseline monitoring enabled determination of fluctuations in turbidity of receiving waters associated with tidal phases (neap, spring), tidal cycles (flood, ebb) and sea states (calm, slight, moderate, rough, very rough). This information was also used to derive key habitat tolerances.
Modelling and trigger values: To predict the turbidity concentration
that could be generated by the construction process to meet key habitat tolerances, the results from the
baseline study were incorporated within a diffusion model of plume mixing and dispersion ( Ridd
et al., submitted). The outcome of this model was a set of warning and shutdown trigger values for
pre-discharge and shutdown trigger levels for receiving waters.
Validation studies: The efficacy of the trigger values was validated by deployment of turbidity loggers at fixed locations on adjacent reef slopes.
Compliance monitoring: The model-derived trigger values (Tables 1 and 2) were applied in pre-discharge and receiving water quality compliance-monitoring programs. Compliance was determined by comparing turbidity to the appropriate warning and shutdown trigger values. Raw turbidity data (n=10 per station) were transformed and the 95% confidence interval of the mean was used to determine compliance with trigger values.
Table 1. Warning and shutdown turbidity trigger values (ntu) for pre-discharge
waters
Tidal Cycle |
Tidal Phase |
Trigger |
Sea state |
Calm |
Slight |
Moderate |
Rough |
Very Rough |
Flood |
Neap |
Warning |
4 |
11 |
21 |
43 |
85 |
Ebb |
Neap |
Warning |
6 |
14 |
28 |
56 |
113 |
Flood |
Spring |
Warning |
13 |
32 |
64 |
128 |
255 |
Ebb |
Spring |
Warning |
17 |
42 |
84 |
169 |
338 |
Flood |
Neap |
Shutdown |
17 |
43 |
85 |
170 |
340 |
Ebb |
Neap |
Shutdown |
23 |
56 |
113 |
225 |
450 |
Flood |
Spring |
Shutdown |
51 |
128 |
255 |
510 |
750 |
Ebb |
Spring |
Shutdown |
68 |
169 |
338 |
675 |
750 |
|
Table 2. Shutdown trigger values (turbidity, ntu) for receiving waters
Location |
Trigger |
Sea state |
Calm |
Slight |
Moderate |
Rough |
Very Rough |
Receiving Waters |
Shutdown |
6 |
15 |
30 |
60 |
120 |
|
Routine biological monitoring - Coral Condition Monitoring Program: A
Coral Condition Monitoring Program was implemented to provide validation of the PMP. A detailed description
of this program will be published elsewhere ( R.J. Jones, pers. com.). In summary,
monitoring commenced with a pre-construction baseline survey of coral condition and was conducted routinely
for the duration of the de-watering process. Transects were located at impact and control stations to
monitor 700 tagged coral colonies from four species: Acropora latistella, A. subulata, Montipora aequituberculata
and Turbinaria mesenterina. Each coral colony was routinely assessed for changes in % bleaching
and % partial mortality to ensure warning or shutdown habitat trigger values were not exceeded. To determine
compliance with warning and shutdown trigger values, data were analysed using a Cumulative Frequency Analysis
and Decision Curve Analysis ( Stafford-Smith et al., 1994).
|