MAPPING TROPICAL SEAGRASS BEDS WITH AN UNDERWATER REMOTELY OPERATED VEHICLE (ROV)
Masumi Yamamuro1, Kiyokazu Nishimura1, Kiyoyuki Kishimoto1, Ken Nozaki1, Ken Kato1, Akira Negishi1, Kenji Otani1, Hirofumi Shimizu2, Takeshi Hayashibara2, Motohiko Sano2, Motoya Tamaki2 and Kouki Fukuoka2
1National Institute of Advanced Industrial Science and Technology
Tsukuba, JAPAN
m-yamamuro@aist.go.jp
2Ishigaki Tropical Station
Seikai National Fisheries Research Institute Fisheries Research Agency
Ishigaki, Okinawa, JAPAN
ABSTRACT
The distributions of tropical and subtropical seagrass beds have not been properly monitored in many countries, owing to the limitations of observation methodologies. Conventional aerial photography fails to detect small-sized species and deep-water seagrass beds. We have developed a new mapping system that uses a remotely operated vehicle (ROV) equipped with an underwater digital camera to monitor seagrass beds. This system enables us to take digital photographs of seagrasses delimited by a quadrat frame. With semi-automatic processing of these photographs, seagrass leaf coverage can be calculated.
INTRODUCTION
Seagrasses are flowering plants that have evolved in seawater. There are about 60 species of seagrass in the world (Short et al., 2001). Of the 17 species found in Japan (Kuo et. al., 1995), 10 can be found in subtropical coastal areas. Seagrass beds are among the most productive ecosystems in the ocean, being a major food source for a number of aquatic grazing animals, such as dugong and turtles.
Many problems exist for the long-term survival and health of seagrass in the world's coastal zones. Anthropogenic pollutants have contributed most to the decline of seagrasses around the world, while careless land development has increased suspended sediments in runoff, which reduces light necessary for the growth of seagrass. Loss of seagrass habitat will result in loss of productivity in marine ecosystems as well as the extinction of species that depend on seagrass for survival. Such disturbances are expected to increase along the subtropical and tropical coasts of Asia, including Japan, owing to increased economic development.
In Japan, seagrass beds have been mapped with conventional aerial photography (e.g., Environment Agency, 1994). In the temperate coastal zone of Japan, seagrass beds are mostly composed of eelgrass (genera Zostera), and most of them are big enough to be mapped with aerial photography. For example, the leaf length of the most common eelgrass, Zostera marina, is greater than 1 meter. On the other hand, subtropical and tropical seagrass beds often consist of small sized species, such as Halophila spp., with leaf sizes of about 2-3 centimeters. It is impossible to detect such small seagrasses with aerial photography. Because of such methodological limitations, the Japanese national seagrass-monitoring program reported the area of subtropical seagrass beds to be 1275 ha in 1978 and 6902 ha in 1989, although the decline of seagrass beds was obvious, owing to the development of coastal areas (Environment Agency, 1994). The report attributed the error to incorrect classification of the aerial photography.
In relatively shallow water (<10 m), divers have directly carried out underwater mapping of seagrass leaf coverage by putting down a quadrat of either 1 m2 or 0.25 m2 on seagrasses and estimating the percentage of coverage in situ (Duarte and Kirkman, 2001). To standardize the estimation of seagrass coverage, the divers carry photographs representing 1% to 100% coverage. If a submersible camera is available, diving time can be minimized by photographing the site vertically (including a scale) and estimating the seagrass coverage back in the laboratory (Duarte and Kirknan, 2001). Seagrass coverage is estimated by the naked eye.
For mapping deepwater seagrass meadows (>10 m), on the other hand, it has been recommended that an underwater video camera and GPS (Global Positioning System) receiver be used, because the working time of divers is restricted at such depths (McKenzie et al. 2001). Although using a digital video camera provides a great deal of picture-based information, it is difficult to estimate the seagrass leaf coverage with accuracy because of the low pixel resolution. Thus, the information for deep seagrass beds has not been of the same quality as that for shallow subtidal meadows (< 10 m), where divers using quadrats can map seagrass coverage. Moreover, the position of the divers at the bottom cannot be exactly determined by GPS at the surface of the sea.
The aim of this research is to develop a new system of mapping seagrass beds growing in subtidal waters. We use a remotely operated vehicle (ROV) equipped with a submersible digital camera and a quadrat. The underwater position of the ROV is determined acoustically, and we are developing a computer system to analyze the digital photographs to evaluate the seagrass coverage.
SYSTEM SPECIFICATIONS
In coastal areas, position can be determined by GPS and acoustic navigation systems. We used DGPS (Differential GPS) to position the ROV on the water surface. When the ROV is under water, an acoustic navigation system is the only available positioning system. There are two types of acoustic navigation system, the LBL (Long Base Line) system and the SSBL (Super Short Base Line) system, both of which use frequencies of 19 and 20 kHz. In our system, the error of the LBL system and the SSBL system was 1 m and 10 m, respectively, when the distance between the transponder and transducer was 100 m. Thus, we found the LBL system (Fig.1) to be better suited for shallow seagrass beds. We also invented a flexible, rising-and-falling buoy system on which to deploy the transponders to allow their positioning in shallow coastal waters under the influence of large tidal changes. This positioning system allows the ROV to move freely over the seagrass bed for monitoring. The position of the ROV is monitored with a video camera system attached to a moored balloon, and a real-time image is transmitted to a shipboard monitor. This enables us to avoid obstacles in the way of the ROV. A diver holding a digital camera on the other hand, has to swim along a fixed transect line whose position is measured beforehand.
(Enlarge: 42KB) |
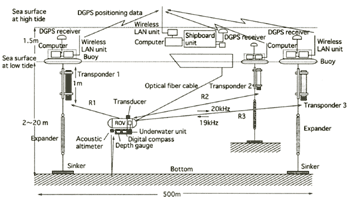 |
Figure 1. Schematic diagram of the LBL positioning system
A rectangular frame was fitted to the ROV to take underwater photographs of the seagrasses at the same camera angle as that used by scuba divers with a quadrat frame (Fig.2, Photo 1). Because the photographs are taken with a digital camera, the digital images are easily transferred to a personal computer for further analysis. The computer filters the image through the green light channel, and the seagrass coverage is calculated semi-automatically by using digital image processing based on the Otsu classification standard (Otsu 1979, Photo 2).
(Enlarge: 50KB) |
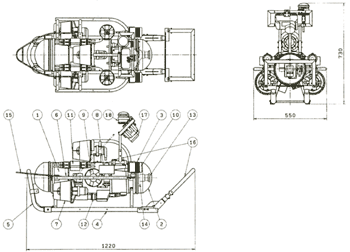 |
Figure 2. Plan of the ROV (1) pressure-resistant box, (2) acrylic dome,
(3) V-retainer clamp, (4) skid, (5) rear bumper, (6) side bumper, (7) horizontal thruster, (8) vertical
thruster, (9) side thruster, (10) light, (11) plug, (12) cable connector, (13) Color TV camera, (14) tilting
apparatus, (15) Vehicle bail, (16) quadrat frame and digital camera frame, (17) digital camera. (18) GPS
Photo 1. ROV equipped with a quadrat frame
Photo 2. Semi-automated calculation of seagrass coverage (%)
FUTURE DIRECTION
Conventionally, underwater video camera systems have been used for ground truthing aerial photography for mapping seagrass beds (McKenzie et al., 2001). However, such systems cannot be successfully applied to deep subtidal areas, because relatively small sized-seagrasses are distributed there. For example, in Florida Bay, Thalassia and Syringodium were found at a maximum depth of 18.0 m, Halodule at 18.6 m, and Halophila decipiens at 26.5 m (Fourqurean et al., 2001). Thalassia testudinum was found even deeper in an area in Cuba (Buesa, 1975). Aerial photography cannot detect such species growing in deepwater areas. Also, the underwater video camera systems for ground truthing may easily fail to recognize such small species, or the video system may not operate at such depths. Because our ROV system uses an acoustic positioning system, a survey line does not need to be fixed beforehand, and the ROV can freely monitor any subtidal area.
There is a lack of information on the status and health of seagrasses worldwide, particularly in the less economically developed regions. Therefore, the UNEP World Conservation Monitoring Centre is presently conducting a global assessment of the status of seagrasses (Green and Short, in press). Local-scale monitoring programs are also ongoing worldwide; these include Seagrass Watch (Australia), Action Plan for Marine Vegetation in the Mediterranean, Caricomp (Caribbean), and Seagrass Net (USA). We would like to propose the development of a standard method for monitoring seagrass beds worldwide using remote sensing technology, including aerial photography, together with our system. Our system can semi-automatically record high-quality digital images, which can be used for later rough identification of seagrass species at sites facing sudden disturbance by human activity.
The present price of the whole system is ca$ 125,000 which is rather expensive for most developing countries. However, our monitoring system can dive to 140 m and can operate for longer than a diver, and the position of the ROV when taking pictures is automatically recorded on hard disk. The digital images may become a valuable testimony of the present status of coastlines, because rapid economic development can dramatically change it in a moment. These merits may offset the demerit of the cost of our monitoring system. International cooperative monitoring projects between developing and developed countries would also be a promising first step toward protecting the valuable seagrass bed ecosystems of the world.
ACKNOWLEDGEMENT
This study was supported by the Experimental Research Budget for National Institutions Involved in Pollution Prevention, Ministry of the Environment, Japan.
REFERENCES
Buesa, R.J. 1975. Population biomass and metabolic rates of marine angiosperms on the northwestern Cuban shelf. Aquatic Botany 1:11-23.
Duarte, C.M. and H. Kirkman. 2001. Methods for the measurement of seagrass abundance and depth distribution. In Global Seagrass Research Methods, edited by F.T. Short and R.G. Coles, 141-153. Elsevier Science B.V.
Environment Agency. 1994. Algal and seagrass beds. Tidal flats, algal and seagrass beds and coral reefs in Japan. Vo1.2. 400 pp.
Fourqurean, J.W., M.D. Durako, M.O. Hall and L.N. Hefty. 2001. Seagrass distribution in south Florida: a multi-agency coordinated monitoring program. In: Porter, J.W. and K.G. Porter, eds. The Everglades, Florida Bay, and the coral reefs of the Florida Keys. CRC Press LLC.
Green, E.P. and F.T. Short, 2003. World Atlas of Seagrasses. Prepared by the UNEP World Conservation Monitoring Centre. University of California Press (in press)
Kuo, J., Z. Kanamoto, T. Toma and M. Nishihira. 1995. Occurrence of Halophila decipiens Ostenfeld (Hydrocharitaceae) in Okinawa Island, Japan. Aquatic Botany. 51:329-334
McKenzie, L.J., M.A Finkbeiner and H. Lorlam. 2001. Methods for mapping seagrass distribution. In Global Seagrass Research Methods, edited by F.T. Short and R.G. Coles, 101-121. Elsevier Science B.V.
Otsu, N. 1979. A threshold selection method from gray-level histograms. IEEE Transactions on Systems, Man, and Cybernetics, 9:62-66.
Short, F.T., R.G. Coles and P. Pergent-Mantini. 2001. Global seagrass distribution. In Global Seagrass Research Methods, edited by F.T. Short and R.G. Coles, 5-30. Elsevier Science B.V.
|