VERIFICATION OF SIMULATED RESULTS
(1) Sea surface temperature and height fields
Since sea surface temperature (SST) field directly reflects a seasonal meridional migration or variation of temperature field affected by small scale eddy activity, we present a snapshot of SST field first in Figure 1a as a concise but informative figure that tells us what our eddy-resolving simulation looks like, while Figure 1b gives a monthly mean observational field of the corresponding month with a horizontal resolution of 1 degree. We see that basin scale SST patterns together with such mesoscale features as the existence of ubiquitous eddy activities, the Kuroshio's meandering and the Gulf Stream paths with the right separation latitudes, Natal pulses in the Agulhas Current and herringbone structures of the Legeckis waves in the eastern tropical Pacific are well reproduced in our simulation.
(Enlarge: 72KB) |
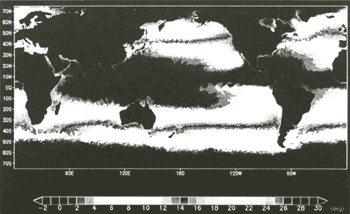 |
Figure 1a. A snapshot field of sea surface temperature in 15 October at
45th model year
(Enlarge: 82KB) |
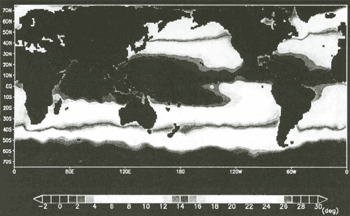 |
Figure 1b. Observational monthly mean sea surface temperature in corresponding
month
Depicted in Figure 2 is the annual mean sea surface height (SSH) field obtained from our simulation. We can clearly see that, consistent with a simple geostrophic relation, the SSH in the subtropics is higher than that in the subpolar region and the lowest SSH appears in the southern flank of the strong Antarctic Circumpolar Current. A zonal band of lower SSH can be noticed along 7°N in the Pacific, which corresponds to the boundary between the North Equatorial Current and the North Equatorial Countercurrent and we can say that the basin-wide pattern of the mean SSH field is simulated well. Note, however, that these features are also well reproduced in numerous other simulations reported so far with much coarser horizontal resolution so that they are insensitive to the explicit representation of mesoscale eddies. One of the intriguing features in Figure 2 is the existence of a fine frontal structure associated with the boundary between the Kuroshio Extension and the Oyashio current systems in the North Pacific.
(Enlarge: 82KB) |
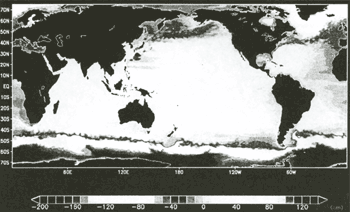 |
Figure 2. Simulated annual mean sea surface height
Three major fronts can be seen to the east of 160°E, two of them merge in the
region extending from 140°E to 160°E and the other corresponds to the sub-arctic front. This fine frontal
structure is similar to the observed one discovered by the satellite AVHRR (Advanced Very High Resolution
Radiometer) infrared imagery (Fig.1 of Mitchell et al., 1996) in which they showed the existence of multiple thermal fronts in the North Pacific aligned in the zonal direction. Using
hydrographic observational data, Mizuno and White (1983) also identified
this bifurcated structure of the former fronts, namely, the Kuroshio Extension bifurcates at 37°N, 163°E
and the northern branch extends along the Shatsky Rise to reach 40°North. This is one of the striking
features that the present high horizontal resolution model successfully simulates. In fact, this frontal
structure was not simulated well by relatively coarser resolution models ( e.g.
Kagimoto and Yamagata, 1997; Mitchell et al., 1996).
Another feature seemingly reproduced well by our high horizontal resolution of the model can be seen in SSH variability fields. Figure 3 shows the root-mean-square variation of the SSH anomaly. The variability maxima (〜40 cm) appear in the western boundary current regions such as the Kuroshio/Kuroshio Extension, the Gulf Stream/the North Atlantic Current, the Malvinas Current and the Agulhas Current and also along the Antarctic Circumpolar Current. For example, in the Kuroshio Extension region, a high SSH variability region extends from Japan coast to the date line in forming a zonal band. This is mostly due to the path variation of the Kuroshio Extension (Mizuno and White, 1983).
(Enlarge: 93KB) |
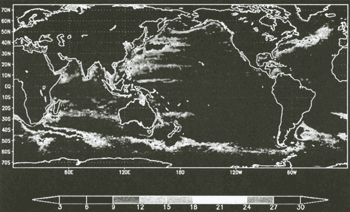 |
Figure 3. Root-mean-square variation of sea surface height anomaly
Fairly high SSH variability regions are also seen along 20°N and 6°N in the
western Pacific. Locations of these high SSH variability regions in the Pacific are observationally confirmed
by the satellite altimetry ( Le Traon and Orgor, 1998; Qiu, 1999). In the North
Atlantic, the region where energetic eddy activities are reflected in high SSH variability extends from
the Cape Hatteras to the Irminger basin. The amplitude of the maximum variability is almost the same as
the observed one ( Le Traon and Orgor, 1998) though its meridional extent is somewhat
broader in the Gulf Stream extension region than the observational counterpart. In short, we may safely
say that the present simulation reproduced observed SSH variability quite well. We think that this success
is partly attributable to the employed high resolution not only in the horizontal ( Smith
et al., 2000) but also in the vertical direction ( Schmitz and Holland, 1986).
|