Stability of Stored Pellets
The biggest concern about mass storage of palletized NGH during ocean transport is dissociation control, or ensuring the stability of the gas contained in the pellets during bulk transport over long periods. With methane hydrate, the equilibrium temperature under atmospheric pressure is about -80℃ (Sloan, 1998). In general, NGH dissociates into gas, water or ice at above the equilibrium temperature. However, a self-preservation effect, by which NGH can be kept at above the equilibrium temperature, was announced by Yakushev et al. in 1992. The mechanism of the self-preservation effect is that when NGH formed under high-pressure and low-temperature is brought back to the low-pressure condition, a part of NGH dissociated on its surface forms an ice membrane on NGH which controls the dissociation of inner NGH. Meanwhile, Ebinuma et al. (1992), have announced that if the NGH is scattered onto ice below freezing point, dissociation of NGH is fairly well controlled even under thermodynamically unstable atmospheric pressure conditions, implying the possibility of NGH transport at storage temperatures as high as -15℃elsius. Applying this self-preservation effect, experimental studies were carried out to determine the feasibility of securing stable storage of pelletized NGH over long periods under atmospheric pressure in ten Centigrade minus numbers. Experimental equipment was an 11 ml container filled with pelletized NGH with a pinhole (ψ0.8 mm) to eliminate pressure buildup, and the stability of the pelletized NGH was determined by measuring changes in its weight, as a reduction in weight was attributable to gas dissociation. The two-week experiment was to simulate bulk ocean transport of pellet NGH by a carrier ship.
Figure 7 shows the measured weight changes of powdered and pelletized NGH stored at a temperature of -20℃elsius. As can be seen, Figure 7 shows that the pelletized NGH is far more stable than powdered NGH, as pelletized NGH gas dissociation under these conditions was below 0.25wt%/d of total gas weight, which is stable enough for practical use, because this dissociation rate is almost equal to the boil off gas rate of LNG carrier. Figure 8 shows the change in dissociation rate over time at different storage temperatures. Stability due to self-preservation is well maintained between -20℃ and -15℃, but a sharp increase in gas dissociation is evident at minus 5℃elsius. Accordingly, it is suggested that NGH pellets formed using the techniques reported herein should be stored at below -15℃elsius.
Figure 7. Respective dissociation rate of pellet and powder
Figure 8. Relation between storage temperature and dissociation rate
Gasification Characteristics
As noticed from the self-preservation effect, NGH, when dissociated, makes an endotherm taking heat from the circumference, which means that the NGH itself will be cooled down and gas dissociation eventually decreases. When the gas contained in NGH needs to be utilized, the pellets it need to be heated to accelerate the gas dissociation, the need to respond quickly to changes in gas demand may sometimes require considerable heat energy. Our experiment assumes that the pelletized NGH would be gasified rather rapidly and therefore gasification characteristics were investigated with gasification temperature and pellet size as experimental parameters. Figure 9 shows gasification experimental equipment. Experimental conditions were as follows: Pellets were all of ψ20mm. Several pellets were put simultaneously into a gasification tank. Gasification was achieved by heating the water in the tank through the tank shell using a thermostat. The gasification pressure was set at 3.6MPa assuming pressure feeding of gas by pipeline.
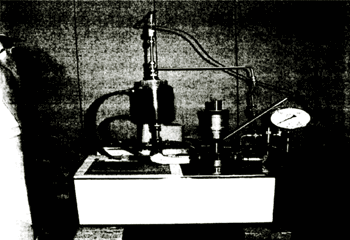
Figure 9. Gasification test equipment
Figure 10 shows how gasification rate changes at 3.6 MPa according to the differences between NGH equilibrium temperature and gasification temperature. Figure 11 shows a comparison of gasification rate between pelletized and powdered NGH by size simulation. Figure 10 shows that gasification gas increases in proportion to the temperature difference between the pellets and the heat medium. This is because calorific transfer to the pellets differs with temperature difference. Figure 11 shows rapider gasification rate for the powdered NGH than for the pellet, which is believed due to differences in surface area to the weight. The pellet gasification rate depends on and is limited by the heat transfer efficiency between the pellets and the heat medium. The experiment also suggests that the adjustment of pellet size by crushing will increase the pelletized NGH gasification
Figure 10. Gasification rate with ΔT (ΔT: Difference between the equilibrium
temperature and gasification temperature)
Figure 11. Gasification rate of pellet and powder
CONCLUSION
The development and confirmation of the effectiveness of the various component technologies in our study has proved that the long distance transportation of natural gas by pelletized NGH is technically quite feasible. MES is now building a pilot plant combining each component of the process such as production, pelletizing, storage, transportation in bulk and gasification into one continuous system. Upon completion of the plant, we will collect more engineering data and scale-up data of the system through various tests and operations and further will proceed to an economic feasibility study. It is our intention and commitment to realize a system for natural gas transport by the Pelletized Natural Gas Hydrate in the near future.
ACKNOWLEDGEMENTS
We thank the Hokkaido Center of the National Institute of Advanced Industrial Science and Technology for their enormous contribution to this project.
REFERENCES
Ebinuma, T. and N. Maeno. 1992. Mechanical behaviors of polycrystalline ice
containing pressurized gas enclosures, Physics and Chemistry of Ice, Hokkaido University
Press, Sapporo, Japan.
Gudmundsson, J.S. and A. Borrehaug. 1996. Frozen hydrate for transport of natural
gas, Proceedings of 2nd International Conference on Gas Hydrate, Toulouse, France.
Sloan Jr., E. Dendy. 1998. Clathrate hydrates of natural gas - 2nd ed., rev. and expanded, Marcel Dekker, New York.
Stern, L.A., et al. 2000. Anomalous preservation of pure methane hydrate at
1 atm, Journal of Physical Chemistry B, 105, 1756-1762.
Yakushev, V.S. and V.A. Istomin. 1992. Gas hydrates self-preservation effect,
Proceedings of Physics and Chemistry of Ice '91 Symposium, Hokkaido Univ., Sapporo, Japan.
|