3. ATLANTIC OCEAN CHANNEL
3.1 Background
Among the major channels that comprise the transportation network providing access to the inner harbor areas of Norfolk and Newport News, the traffic activity of the AOC is relatively less crowded. On the other hand, the AOC is exposed and subject to a wide range of environmental conditions including wind, wave tide, and current. For deep laden vessels, such as a collier, the vertical motions and the risk of grounding are concerns to the ship operators.
During the mid-80's, CAORF conducted a compressed-time (off-line fast-time vs. real-time simulator) study of the AOC for ACOE-ND [4]. The study ship was a loaded 150K DWT collier going outbound. The study analyzed the vertical motions and the probability of grounding for several channel water depths. Presently, large vessels calling on Norfolk Harbor have expanded to include large containerships. Different from the colliers, these containerships could also arrive at the port heavily loaded. The purpose of the current study was to evaluate the vertical motions and the probability of grounding of both a large collier and a Post-Panamax S-Class containership. The study also looked into the vessel controllability issue while transiting in AOC, in the presence of current, wind and wave. The collier served as the baseline ship, while the containership was the primary study ship. This investigation used an off-line approach, and was conducted by the Danish Maritime Institute [2] in parallel with other Norfolk Harbor simulator studies.
3.2 Methodology
Two approaches were used in AOC study: Frequency Domain (FD) and Time Domain (TD):
Frequency Domain Analysis. This approach calculated the grounding probability at six locations on each ship, including combinations of vessel type, speed, transiting direction, water depth, and the occurrence of a given sea-state with directions based on scatter diagrams for the area. It also considered squat, tide, first order ship responses to waves, and the second order ship responses to waves, such as vertical set-down.
In the FD, the computation of the probability of grounding used the linear superposition of the ship motions in heave, roll and pitch. It was assumed that the seabed was flat and even; the wind induced heel was neglected with the assumption that the wind tanks would compensate for it. The sea states were described in a spectral form; and, likewise were the long-term statistics of the sea states, including direction and the tidal height. They were all described by their distribution functions rather than in a deterministic form
Time-Domain Simulation. This approach investigated grounding occurrence and vessel controllability using fast-time simulation. The ship models were 6-DOF maneuvering models. The simulations included the effect of first and second order wave response and the squat effect. A numerical navigator was used to control the ship to sail through the AOC in the presence of wind, current, and waves. The selection of investigated environmental scenarios was based on the results from the FD study, as well as general knowledge of maneuvering ships in confined waters. Ten sample wave functions were used for the single test scenario, to obtain a desired level of statistical information. One part of the results, given as the probability of grounding in the AOC for specific environmental conditions, was compared with the corresponding results obtained in the FD study. The other part of the results, given as the ship controllability performance in the adverse environmental condition, was examined alone.
Due to the intrinsic limitations of the FD analysis and TD simulation, each of these approaches provided only a partial view of the vessel motions. Yet the results from these two approaches complemented each other. Therefore, the data were compared with each other as part of the quality control. They were also reviewed and interpreted in an integrated way to provide a more complete picture, covering both grounding and controllability issues.
3.3 Results
Tables 1 and 2 are sample results of the FD analysis in a condensed form, i.e., the probability of grounding given as the sum of the maximum probability for each of the six points for each wave direction.
where Pi,n(g) is the probability of grounding for a certain test point (i) and a certain wave direction (n) and ndir is the number of wave directions.
Table 3 illustrates the sensitivity of grounding probability to wave height, where wave heights above 3m are excluded, i.e., the ship will not enter the AOC in sea states higher than 3m, corresponding to a down time of 11 days/year. Table 4 is a sample presentation of the statistical results from the TD study for HS=1.5m, Tp=10s, waves and wind coming from the East. Descriptive statistical analyses of rudder and rpm were used to investigate the controllability and safety issue, in accordance with PIANC [5].
Table 1 Grounding Probability - Outbound Collier
Collier |
Out-bound |
Depths[feet] |
Speeds[kn] |
12 |
10 |
8 |
6 |
52 |
1.000 |
1.000 |
1.000 |
0.321 |
54 |
1.000 |
0.433 |
0.209 |
0.148 |
56 |
0.347 |
0.189 |
0.129 |
0.119 |
58 |
0.167 |
0.108 |
0.078 |
0.066 |
60 |
0.099 |
0.063 |
0.055 |
0.053 |
|
Table 2 Grounding Probability - Outbound Container
Container |
Out-bound |
Depths[feet] |
Speeds[kn] |
14 |
12 |
10 |
8 |
52 |
1.000 |
0.406 |
0.250 |
0.208 |
54 |
0.268 |
0.214 |
0.149 |
0.114 |
56 |
0.159 |
0.087 |
0.067 |
0.059 |
58 |
0.064 |
0.050 |
0.038 |
0.030 |
60 |
0.033 |
0.023 |
0.018 |
0.015 |
|
Table 3 Grounding Probability with Wave Heights > 3m Excluded - Outbound Container
P(Hs>3m)=0 |
11days/year |
Container |
Out-bound |
Depths[feet] |
Speeds[kn] |
14 |
12 |
10 |
8 |
52 |
1.000 |
0.377 |
0.223 |
0.181 |
54 |
0.239 |
0.188 |
0.123 |
0.088 |
56 |
0.133 |
0.062 |
0.042 |
0.035 |
58 |
0.040 |
0.027 |
0.015 |
0.009 |
60 |
0.012 |
0.004 |
0.001 |
0.000 |
|
3.4 Conclusions
Based on the comparison between the two vessels, with the collier serving as a reference of an already known situation, the container ship had less probability of grounding than the collier. This was demonstrated by both FD and TD results. This conclusion was expected, due to the smaller draft of the container ship, and also due to the longer hull of the container vessel combined with the restricted water depth - the restricted water depth reduces the wavelength significantly from its deepwater wavelength in the range of wavelengths that stimulate vertical motions on the vessel. Note that the probability from TD was generally higher than that from FD. One reason was that different statistical approaches were used in the FD and TD calculations. Other reasons lie in the different assumptions in the two approaches. In the TD study,
・When the speed differed from target speed, the revolutions were increased, resulting in higher speed until a steady situation was found again.
・The current resulted in higher speed through water when it was opposite to the course of the ship, which would result in larger squat.
・The wind caused the ship to heel, which couples with yaw and thus becomes pitch.
・Couplings with pitch that cause dynamic pitch.
If all sea states with Hs > 3 m have been removed and the speed is kept low, the probability of grounding becomes rather small for the container ship; however, the collier still has some probability of grounding under that situation. Note that for the smallest depth tested, 52', both ships have a noticeable probability of grounding for all speeds.
Based on the analysis of wave directionality effect, it appears that the inbound direction is the more vulnerable direction for the collier, whereas the containership has a slightly higher risk of grounding in the out-bound direction, than in the in-bound direction.
Based on the results of the TD simulation, it is concluded that the containership is generally easier to control than the collier in the AOC, when in the presence of 30 knots wind from the East, a uniform 0.75 knots current going south, and the tested wave conditions.
Table 4 TD Results in Relation to Speed and Depth
3.5 Adopted Measures
Based on the AOC study, the ND adopted the following measures to address the identified issues:
Vessels operating in the AOC are subject to larger motions than in the TSC and NHR channels and require a deeper channel depth in the AOC. A 52' channel depth was chosen as project depth. A result of the AOC study was a series of tables relating percent probability of grounding versus channel depth. The ND analyzed this information with construction cost data to develop a series of probability of grounding versus construction cost graphs. Combining this information with other navigation and dredging considerations helped the ND determine that 52' was the appropriate project depth.
This channel has never been dredged before for navigation and only recently spot-dredged as a borrow site for a beach nourishment project. The ND incorporated the simulation study with other considerations to determine the appropriate construction depth. The ND determined dredging depths of 53' were required plus one foot allowable overdepth. The contractor will be required to dredge the channel to 53 ' and allowed one additional foot of overdepth.
The simulation study assisted in the economic evaluation of the channel. The ND was responsible for evaluating the economics of the channel deepening project. The engineering staff at the ND used the baseline condition simulation data with other information to determine how existing traffic, would benefit from a deeper channel. This helped to provide additional economic justification for the channel deepening project.
4. NORFOLK HARBOR REACH
4.1 Background
The third element of the 50' Inbound Channel slated for improvement is the NHR, which is currently maintained by the ACOE as an asymmetric channel, a 650' wide outbound element is maintained to 50' deep, and a 600' wide inbound element is maintained to 45' deep. A channel design consideration was to move the western channel boundary inward 250', to change the channel width from 1,250' to 1,000', and for incremental deepening of the inbound side of the channel to 50'.
The purpose of this study was to evaluate this 1,000' wide NHR Comparative Test Channel Configuration (CTCC), when used by deep draft inbound containerships. The CTCC was tested to determine if a reduction in width would be feasible. Such a reduction in width would reduce the quantities to be removed from the channel with the future dredging contracts. This reduction in quantity would save the Corps and the local sponsor significant funds on the 50' Inbound Channel deepening project.
4.2 Methodology
This study used a man-in-the-loop simulation approach. It was conducted using both the CAORF full-mission VBSS and the Second Bridge in an interactive mode. The evaluation was based on comparing the simulated transits of the fully loaded S-Class ship (as the new design vessel) in the CTCC (as the new/modified design channel); with those of a partially loaded S-Class ship that approximates the largest containership in existing vessel traffic (as the baseline vessel), in the existing NHR channel configuration (as the baseline channel). Transits in this baseline vessel provided a standard of acceptable performance, against which the modified design was compared.
Six pilots, members of the Virginia Pilot Association, participated in the study during test runs. Two pilots were simultaneously active in each test scenario, one on each of two simulated ship bridges, which involved a meeting situation in the NHR. One pilot controlled the inbound ship (i.e., fully- or partially-loaded S-Class ship), while the second pilot controlled a fully loaded outbound collier or an S-Class ship. Questionnaire data were collected from the pilots before, during and after the series of simulated transits, to augment the simulator-generated data.
A series of 36 simulated transits were conducted. The scenarios were designed to address the pilots' concern of meeting near the turn with the Norfolk Harbor Entrance Reach (NHER), in the presence of a worst credible 50-knot West wind. Figure 5 illustrates an example of the difficult meeting situation.
Fig.5 Focus of NHR Study Gaming Area.
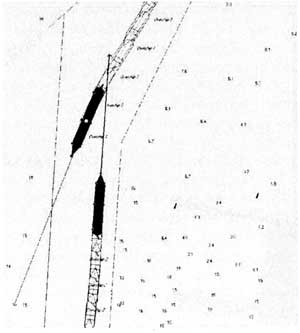
Similar to the TSC study, the simulator experiment began with a series of validation and filtering runs. A problem of the simulator stopping was often encountered during these runs, due to the grounding of either inbound or outbound vessels. Note that the 50' draft collier was sailing in the outbound channel of a nominal 50' depth. The simulation depth database does reflect the actual water depths in macro scale, i.e. a few feet deeper than 50', but not evenly. However, the clearance is too small to allow significant squat. In the real world, the collier glides over the muddy bottom and the vessel experiences gentle vibrations (as stated by the pilots). On the simulator, a negative DUKC creates a math model error message, stopping the simulation. The 3' UKC for the loaded S-Class vessel does not allow much speed before the ship touches the bottom, even without considering the vertical motions induced by the rudder, passing ship effects, and wind. It was decided to set the simulator to a conventional maneuvering mode (surge, sway, and yaw only) for this study, and note the vertical motion issues in the findings, conclusions, and recommendations.
4.3 Findings
The test transits under the worst credible conditions were extremely difficult. Meaningful problems were encountered in about half of the transits, with over 15 % resulting in grounding outside of the channel or a collision. Most of the problems occurred with the inbound ship. As expected, most problems occurred during the earlier runs of each set, consistent with the expected learning effect that typically accompanies simulator research. Nevertheless, the problems were also consistent with differences in the channel/ship configurations.
Comparison of Partially Loaded and Fully Loaded inbound S-Class Ships. The two inbound S-Class ship configurations (38' and 47' draft) had similar conditions in 12 transits, specifically when meeting the outbound collier in the 1,000' wide CTCC. Only minor, and non-significant, differences were found between these ship configurations on several objective measures of performance, such as the range between the inbound and outbound ships at the closest point of approach (CPA). A substantial difference was found, however, in the number of channel excursions made by the two ship configurations: The 38' draft S-Class ship made twice as many channel excursions as the 47' draft ship, touching or going outside of the channel in two-thirds of the transits. However, the 38' draft ship never grounded, because sufficient water depth existed outside the channel (Note, pilots indicated that it is not unusual to go outside of the channel in the area of the study, to initiate or recover from a turn, especially when under extreme conditions). In contrast, the 47' draft ship was much more constrained by the channel boundaries. It made only half the number of channel excursions, but, ran aground both times it went out of the channel. Although the two S-Class ship configurations performed somewhat similarly, the navigation risk was greater for the 47' draft ship under the extreme wind conditions.
Comparison of Baseline Channel and CTCC. The multiple analyses provided consistent results indicating that the baseline channel configuration resulted in better pilot-ship-waterway performance than the CTCC. Results supporting this finding included:
・Significantly fewer navigation problems were experienced in the 1,250' wide baseline channel.
・The ship-ship safety margin at CPA was significantly larger in the 1,250' wide channel. This was accomplished while achieving the same average channel safety margin as achieved in the 1,000' channel.
・The average ship-ship safety margin at CPA in the 1,250' channel exceeded the goal expressed by 5 of the 7 pilots, as compared with the goals of only 2 of the 7 pilots in the 1,000' channel.
These findings provide evidence that the 1,000' wide channel would present a higher risk of collision than the existing 1,250' wide channel.
NHR Channel Depth. The limited channel depth was one of several factors that contributed to make the test scenario particularly difficult. Very shallow UKC, such as that of a 47' draft ship in a 50' deep channel, may result in unpredictable ship behavior. In addition, a substantial risk exists for the 47' draft ship to ground inside the channel. The nominal 3' UKC would be reduced by a combination of speed-induced squat, and roll due to high winds, rudder forces and passing ship effects. Most pilots judged the depth to be adequate; half, however, recommended a deeper channel to offset ship control problems, especially in the area of the turn.
Safety of Navigating in the CTCC. The six test pilots judged the 1,000' wide NHR channel as too narrow for safe navigation of two-way traffic, under the conditions investigated in this study. They also stated that the 1,000' wide channel would be less safe than the 1,250' wide channel. When asked if the 1,000' wide channel was adequate, five of the six test pilots said "No". The sixth pilot stated that the 1,000' wide channel would not be adequate in a particular segment of the channel.
The performance data generated from the simulator demonstrated that narrowing the NHR from 1,250' to 1,000' would adversely affect large laden ships. The influence, however, may be less substantial for lesser-draft ships since the water outside channel boundaries will remain at existing depths (although these areas would not be maintained). Pilot opinions, coupled with the pilot-ship-waterway performance data generated by the simulator tests, indicate that a 1,000' wide channel would be less safe than the existing channel for large deep-draft ships.
4.4 Conclusions
The conclusions stated below pertain to the transit conditions described above, unless otherwise noted. The focus of the study was on the inbound ship. The conclusions generally pertain to the inbound ship, unless identified as such.
Difficulty of Navigating the NHR Under Worst Credible Conditions. The simulated transits in the NHR, under the worst credible scenario conditions created in this study, were difficult for the pilots. Their judgments corroborate this conclusion, as do the pilot-ship-waterway performance data generated during the simulated transits. Most of the problems, although not all, occurred with the inbound ships and involved touching the channel boundary, or going outside of the channel without actually grounding. The results clearly demonstrated the challenge of navigating large deep-draft ships in relatively narrow channels under difficult wind conditions.
Safety of Navigation in the 1,000' Wide CTCC. The study provided reasonably compelling results demonstrating that the existing baseline NHR channel configuration was meaningfully superior to the 1,000' wide CTCC. The narrowing of the 1,250' width to a width of 1,000' would significantly reduce the safety of navigation. This increased level of navigation risk would exist for the large ships that currently enter the port as approximated by the 38' draft S-Class ship, and would be even greater for the proposed large deep-draft ships represented by the 47' draft S-Class. This conclusion is reasonable, in view of the fact that the CTCC has 20% less width than the existing 1,250' channel. The results provide evidence that the CTCC would present higher risks of collision and grounding than the existing channel.
The bottom-line conclusion may have been best expressed by one pilot, who stated that the turn into the 1,000' wide NHR would not be a problem if other traffic was not present, even with the severe wind. However, a 1,000' wide NHR would be too narrow for two-way traffic under severe wind conditions, especially in the area of the turn from the NHER.
4.5 Recommendations
Based on the findings and conclusions, the following measures were recommended:
1. Additional means should be investigated to reduce the increased risk to navigation if the channel width were to be reduced.
2. Keep the NHR Channel 1,250' wide.
3. Dredge high spots on the north side of the NHER Channel, to enable ships to use that area to setup for the turn, when necessary.
4. Dredge the Naval Maneuvering Area, to enable ships to use that area when completing an inbound turn, when necessary.
5. Dredge NHR deeper than 50'.
6. Institute practices/procedures and criteria to offset the increased risk to navigation from a narrowed channel.
7. Evaluate risk mitigation alternatives for deep draft ships.
4.6 Adopted Measures
Based on the simulator study, the ND decided to keep the current 1,250' channel width. In addition, the ND adopted the following measures to address the identified issues:
A 50' channel depth was chosen as the project depth. The ship simulation study showed that the design vessel required a 52' deep channel. For reasons similar to the TSC design rationale, the ND determined the project depth to be 50'.
The ND incorporated the simulation study into the design to determine the appropriate construction depth. For the construction contract, dredging depths of 52' will be required, with one foot allowable overdepth. Dredging records for this channel are extensive since the channel is dredged annually. The contractor will be required to dredge the channel to 52', but will be allowed one additional foot of overdepth.
Channel bend wideners were incorporated into the design. The simulation study revealed that the design vessel would benefit from having more maneuvering area near the turn between Norfolk Harbor Entrance Reach and NHR. The ND incorporated channel bend wideners on the northern and western sides of the turn.
|